A story of a star - Part I
We are to embark on a journey to discover the evolution of a star, from its birth in the molecular nebula to its final destiny: a violent explosion, a lasting dimming, a shrunken vessel.
Chasing the Sun
Looking into the heavens, we could be tempted to assume that stars don’t change. Yes, there is a change in the sky season after season, but that may be due to the motion of the Earth around the Sun.
The Sun looks like a stable, unaltered, never-changing object in the sky. But with the advent of astronomical observations, and therefore with the advent of the telescope, the enhanced observation of the Sun showed that the Sun has spots (sunspots) : the stars were changing.
The history of Solar and especially the sunspot’s observations is very intriguing: it appears for the first time in written text in the I Ching (the Books of Changes), a Chinese divination text written in the late 9th century BCE1, where it’s found
a dou is seen in the Sun
a mei is seen in the Sun
where dou and mei are darkening or obscuration.
Also in ancient Greek, around 300 BCE, Theophrastus mentioned, first in the West, this type of event. Then other intellectuals (whether they be proper astronomers, monks, jurists, or historians) noted or cited this strange phenomenon.
The first observation of a sunspot with a telescope was made by Thomas Harriot, an English astronomer, on 18 December 1610. On 9 March 1611, Frisian Johann Goldsmid (latinised Johannes Fabricius) also observed sunspots.
These two discoveries were shadowed by the claims made by Galileo Galilei and Christoph Scheiner to have discovered these sunspots in 1612. Whoever discovered first between the two astronomers, they were beaten (without knowing it) by Harriot.
Where we came, there we’ll go
This brief introduction served to describe the realization that came with the discovery, thanks to the telescope (that rendered unlikely to infer that the sunspots were instead planetary transits), of little changes in the structures, or at least the appearances, of our star the Sun.
We will now delve into minute details in order to understand how stars are made, how they evolve, how they change, and ultimately how they die.
We could say that the evolution of stars is somewhat cyclic.
Stars are formed from Interstellar Medium (ISM) and during their life they expel much of their mass back to the ISM itself, even returning completely to the ISM in the case of Supernovae explosions.
The dominant component (approximately 70% of the mass) of the ISM is hydrogen gas, in the form of neutral hydrogen (HI), ionized hydrogen (HII), and molecular hydrogen (H2). The other 30% comprises essentially helium; metals2 account for a few percent of the total.
ISM presents in different ranges of density, masses, and temperatures, mostly because of the different ways for the hydrogen gas.
In clouds of gas where the hydrogen is primarily atomic, also called diffuse HI clouds, with the following properties:
temperatures vary from 30 to 80 K;
densities between 100 and 800 million particles per cubic meter;
masses between 1-100 solar masses3.
When in these clouds one can find molecular hydrogen in the inner regions, these are called diffuse molecular clouds, with
temperatures vary from 15 to 50 K;
densities between 500 million and 5 billion particles per cubic meter;
masses between 3-100 solar masses;
these clouds measure several parsecs4.
There are also Giant molecular clouds, huge complexes of dust and gas with
temperatures typically of 15 K;
densities between 100 and 300 million particles per cubic meter;
masses between 100’000 solar masses (up to 1 million solar masses);
these clouds measure 50 parsecs.
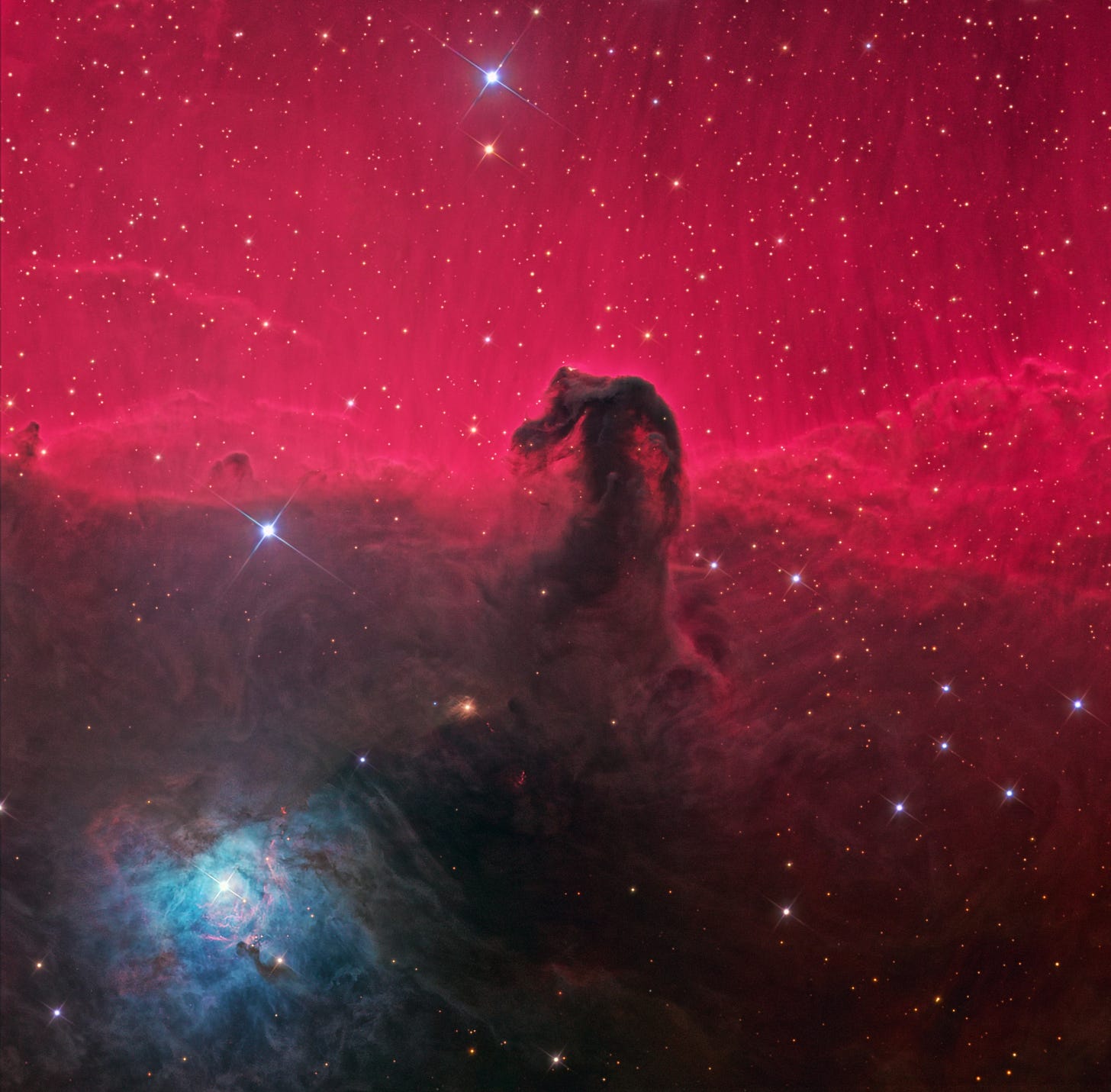
The grand structure of the Giant Molecular Clouds is often divided into superdense regions, like dark cloud complexes (10’000 solar masses, density of 500 million particles per cubic meter, temperatures of 10 K, scale of 10 pc); clumps (30 solar masses, density of 1 billion particles per cubic meter, temperatures of 10 K, scale of a couple of pc); dense cores (10 solar masses, density of 10 billion particles per cubic meter, temperatures of 10 K, scale of a 0.1 pc); hot cores (10 to 3000 solar masses, density of 10-10’000 thousand of billion particles per cubic meter, temperatures of 100 to 300 K, scale of 0.05 to 0.1 pc).
Outside of large molecular clouds, there are clouds of almost spherical shape known as Bok5 globules, with densities of over 10 billion particles per cubic meter, masses from 1 to 1000 solar masses, temperatures around 10 K, and sizes of less than 1 pc.
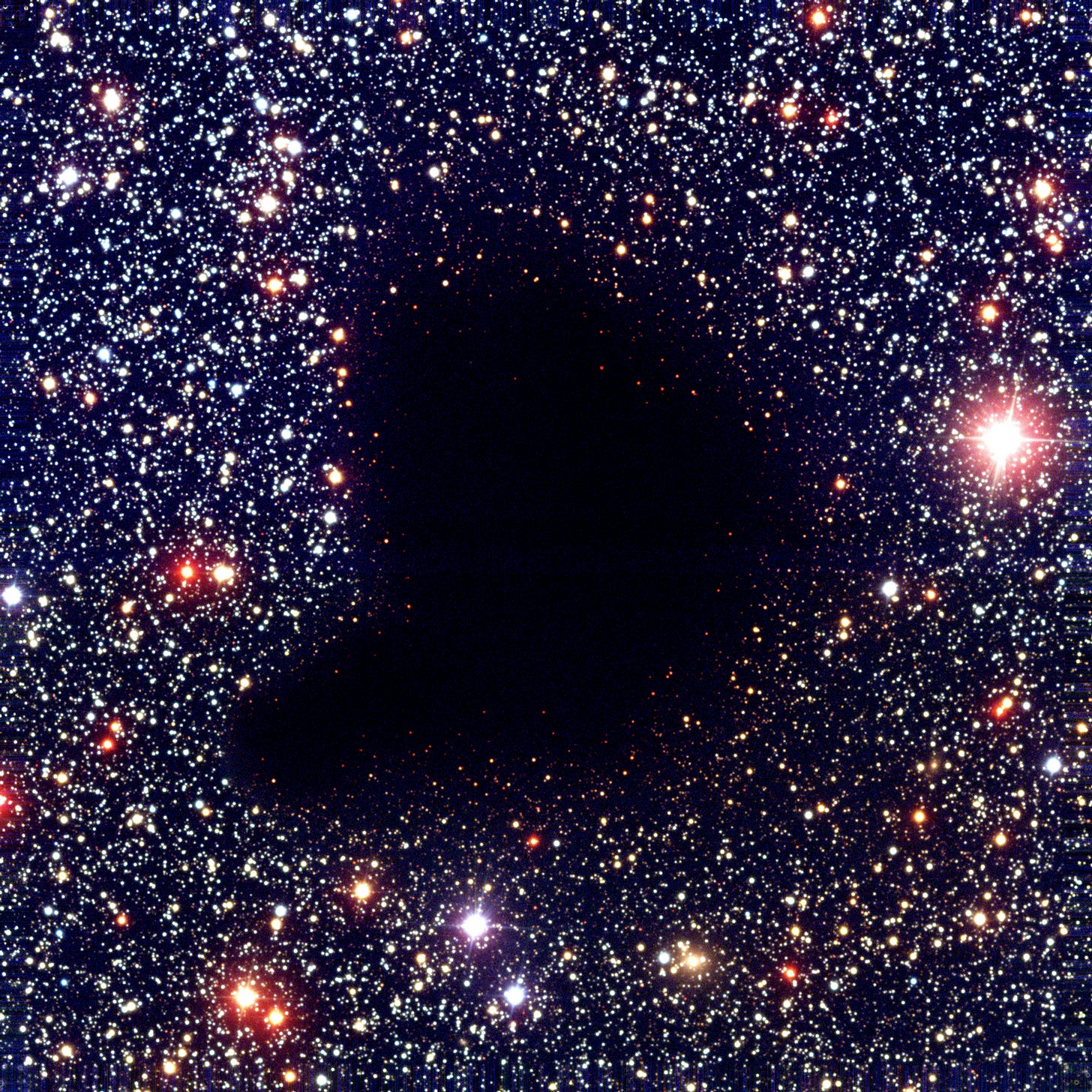
How does the temperature change in all these clouds? Much of the temperature rise comes from cosmic rays, mostly charged particles that come from supernova explosions and that heat the ISM in the clouds6.
The formation of Protostars
The question we would like to tackle now is the following:
what are the conditions that lead to the collapse of the globules and cores in order to create the so-called protostars?
One way to describe this was found by English Astronomer Sir James Jeans, who considered what would happen if a star that doesn’t rotate, not affected by galactic magnetic fields, and without turbulent motion, deviated from hydrostatic equilibrium. The concept used is very simple: if there are two main sources of motion, gravitational potential energy and kinetic energy of the molecular clouds, these two energies will compete to dominate; if the former dominates, the cloud will collapse, but if the latter prevails, the cloud will expand7.
Now all it takes is to understand what is the value of these energies.
If one assumes the cloud of spherical shape, and of constant density, then the gravitational potential energy is approximable to
where G is the gravitational constant, M is the mass of the cloud, and R is its radius.
The kinetic energy can be estimated to be
where N is the total number of particles that compose the cloud, k is the Boltzmann constant, and T is the temperature. The number of particles is derived from the mass of the cloud, divided by the mass of the hydrogen atom and by the mean molecular weight (that is a quantity used to describe what kind of elements compose the cloud).
When equating these two relations one can find the so-called Jeans criterion, both for mass and for size, that decides the maximum mass and the maximum radius the cloud can have in order to not encounter collapse. You can, of course, view them as the minimum mass and radius in order for the cloud to collapse (your choice).
Let’s report an example from BoB8: for a cloud with a temperature of 50K, entirely composed of neutral Hydrogen and a number density of 500 million particles per cubic meter, the minimum Jeans mass for collapse is 1500 solar masses.
But this value is many times the maximum observed mass of the HI clouds: this means that these clouds are stable against gravitational collapse.
The situation may be somewhat different for a giant molecular cloud, with a temperature of 10K and a number density of ionized hydrogen of 10 billion particles per cubic meter. In this case, the Jeans mass for collapse is 8 solar masses, similar to the order of 10 solar masses for these objects: that’s why GMCs are possible sites of star formation, being unstable against gravitational collapse.
What will happen?
In the next chapter, we will go on tackling the collapse of unstable molecular clouds and their fragmentation, and follow the first step of the protostar.
Stay tuned!
Some links for further reading:
https://web.archive.org/web/20060301083022/http://web.hao.ucar.edu/public/education/sp/great_moments.html
https://articles.adsabs.harvard.edu/cgi-bin/nph-iarticle_query?bibcode=2007JBAA..117..346V&db_key=AST&page_ind=0&data_type=GIF&type=SCREEN_VIEW&classic=YES
https://web.archive.org/web/20150102174227/http://www.hao.ucar.edu/education/TimelineB.php
See you soon! (and if you liked subscribe!)
Before Current Era; also known as Before Christ. We will stick to BCE and CE (Current Era, instead of AD).
In Astrophysics, metals are all the chemical elements that are not Hydrogen or Helium.
Bok globules are named after Bart Bok, a Dutch-American astronomer who studied these objects (https://ui.adsabs.harvard.edu/abs/1947ApJ...105..255B/abstract).
Reactions that occur between protons (CR protons) and hydrogen atoms are listed below:
When an atom of hydrogen gets ionized, this means that an electron is ejected from the atom, with some of the energy of the initial proton. When the electron interacts with the ISM molecules, the energy gets transferred to the ISM. The energy gets then redistributed and the temperature rises in the cloud.
actually, it’s a bit more complicated than that; the condition for equilibrium is described by the virial theorem, which states that twice the kinetic energy plus the potential energy is equal to zero. Nonetheless, the simple frame still stands.
aka Big Orange Book, aka An Introduction to Modern Astrophysics, Bradley W. Carroll & Dale A. Ostile, Cambridge University Press.