Just a story of a star: Part III
We follow the fate of a cloud that hopes to become a star: it's a heated voyage, with some cool events in the middle, and two collapses.
In the previous episodes we have encountered a giant molecular cloud, the birthplace of hundreds of stars.
Now, we turn our attention to one single spherical cloud, of one solar mass. Let’s see what happens.
Stellar nursery
We start with a free-fall collapse (the one we encountered here and here). Photons1 can travel great distances before being absorbed by “dust”2, so we are in an optically thin environment: this means that the collapse is isothermal. But the density is not homogeneous, hence there is a slight increase of the free-fall velocity in the center than in the outer parts: this is what we call an inside-out collapse). At some point, the density in the center of the cloud becomes high enough3 that the region becomes optically thick4 and the collapse is more adiabatic.
Now in the inner parts of the cloud, the pressure rises, and the adiabatic collapse reduces the rate of the collapse. This region enters hydrostatic equilibrium5 and has a radius of 5 AU6.
Did you come this far? Congratulations, you saw the birth of a protostar.
This object is now observable as a single entity and can be put on maybe the most important plot that a stellar physicist encounters in their life: that is, the Hertzsprung-Russel diagram.
What is it? What does it tell us? Why it is important?
All these questions (that I’m sure you were asking yourselves) will be answered swiftly.
Digression: Hertzsprung-Russell diagram
or, how to draw points and curves on a plot
The idea of putting stars on a plot arrived in the early twentieth century when astronomers noticed that the observed stars varied in luminosities and absolute magnitudes. This led to the idea that stars begin their “life” with a certain amount of “fuel”, that is, material that can be transformed in luminosity and energy, and as they age they cool off, becoming colder and colder.
This idea is nowadays considered wrong; nonetheless, it helped astronomy progress.
In 1905 Danish amateur astronomer Ejnar Hertzsprung (1873-1967) confirmed that there was indeed a correlation between the absolute magnitudes7 and the spectral type8 of a sample of stars. He noticed also that stars with the same spectral type had different magnitudes, i.e. had different levels of brightness.
He named the brighter stars giants because if you consider stars as great converters of energy in luminosity, then if two stars have the same temperature, and the most luminous must also be the bigger one9.
This relation was still not plotted on a graph. Then Henry Norris Russell (1877-1957), who independently arrived at the same conclusion, also naming dwarfs the dimmer stars, published a diagram, initially known by astronomers as “Russell diagram“.
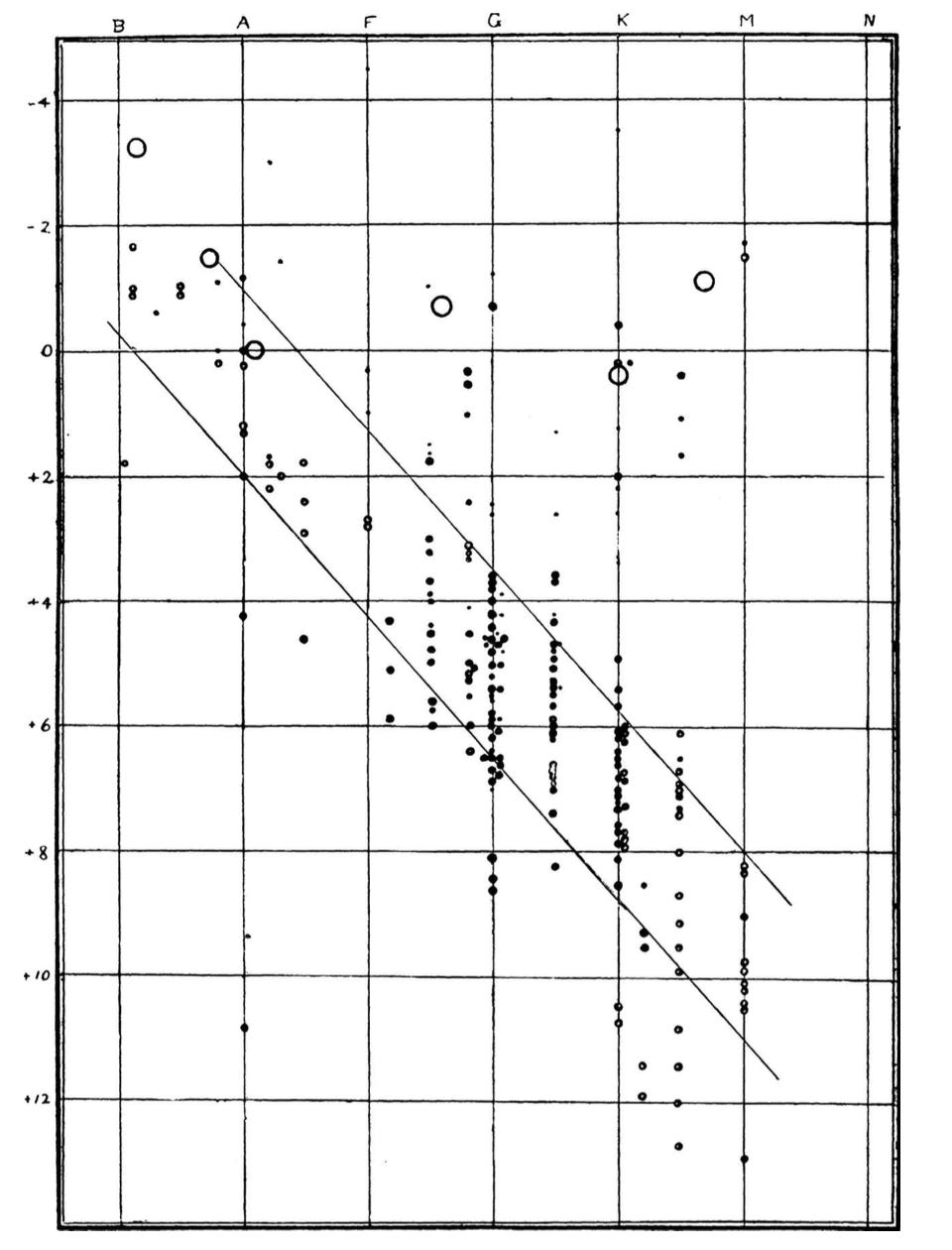
As you can see, most of the points, each representing a star, are displayed between the two solid bands, that define a band called the main sequence, which is the home of the vast majority of stars in the Hertzsprung-Russell (H-R) diagram.
In the lower left, there is one star which is a white dwarf while in the upper right corner, there are the giant stars.
Below there is a newer version of the H-R diagram.
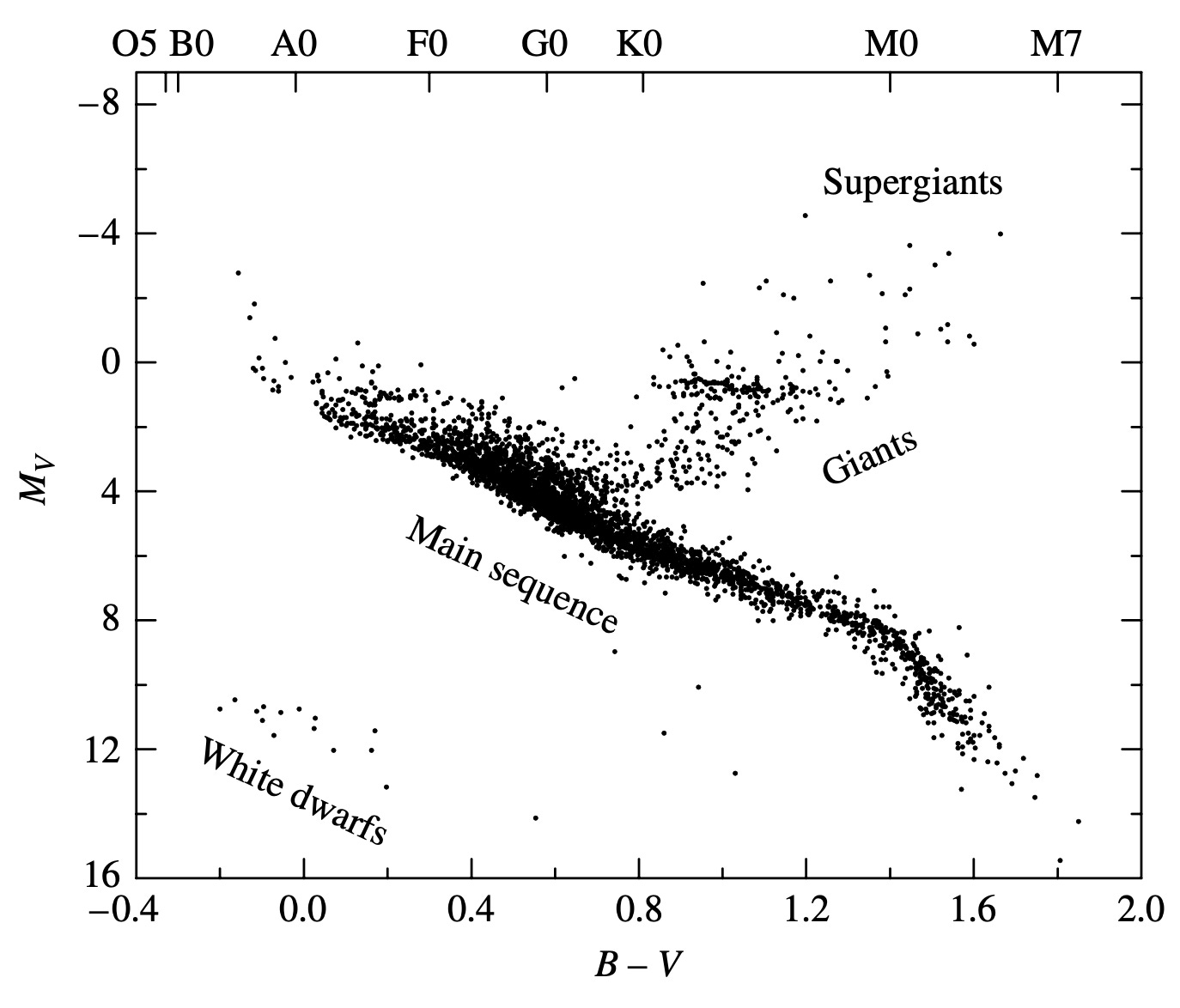
In this second plot you can see the main sequence at the center, and again the white dwarfs in the lower left, with the giants in the upper right.
Let’s return to our protostar.
Our hypothetical cloud resulted in a protostar with a radius of 5 AU, and now we can put it on a H-R diagram. The quantity we need is the luminosity, which can be computed by considering the protostar as a “blackbody”10, and the formula to do so is the following:
Below is displayed the last plot for this episode, which shows the track that different clouds (with different masses) take within the H-R diagram: they all start in the lower right part of the plot, almost parallel to one another.
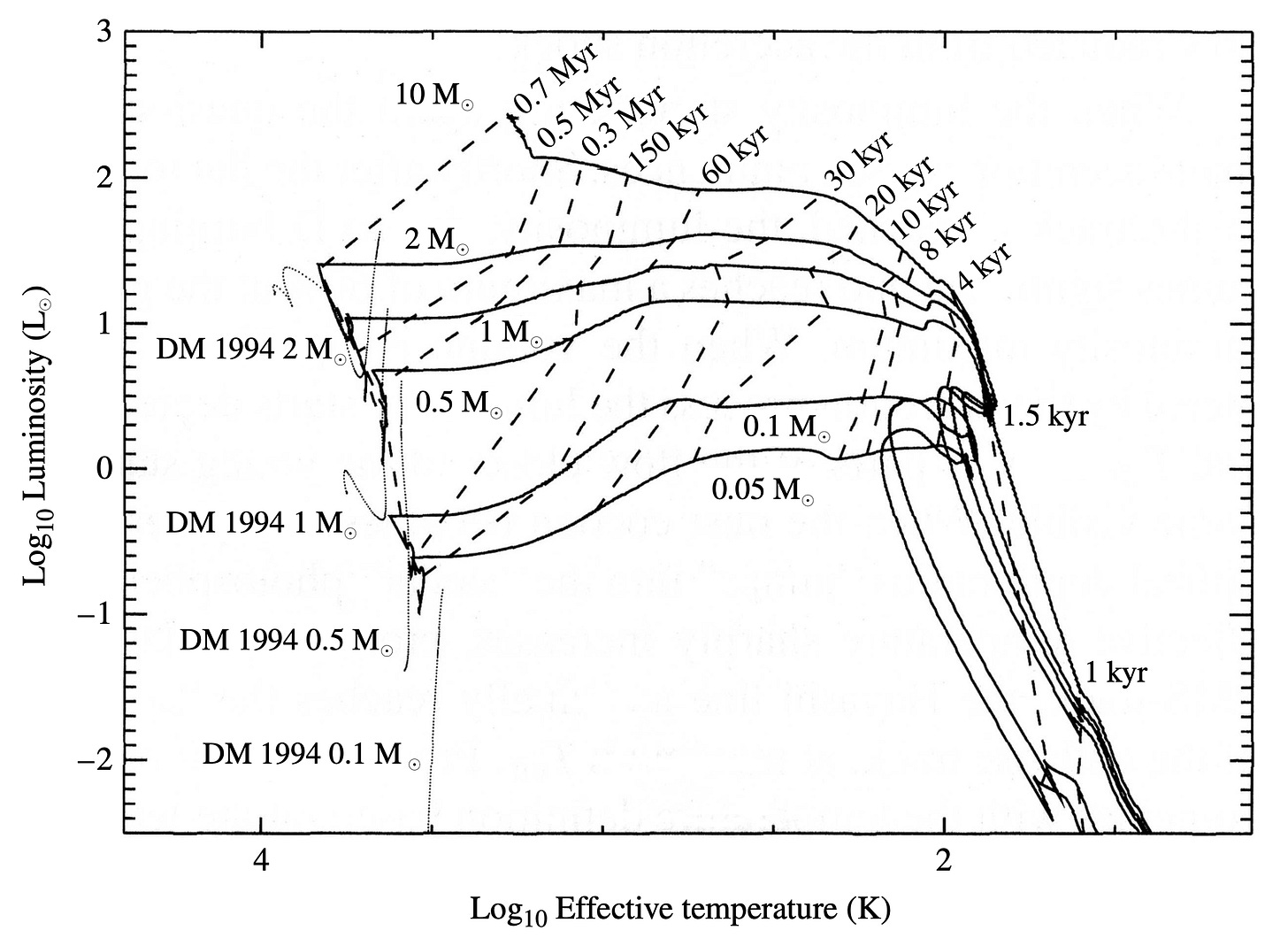
You can see that initially the luminosity increases, as well as the temperature (mind the logarithmic scale and the temperature that increases going from right to left). The collapse continues, and then material above the inner part of the protostar is in free-fall. When said material reaches the core of the protostar, the shock generated by the impact between this material and the core itself generates a lot of luminosity11.
The dust that surrounds the core, when the temperature rises, gets hot and vaporizes. This means that now photons can wander freely out of the cloud: opacity drops and the cloud/protostar becomes optically thin.
Ok, now luminosity and temperature continue to rise. When the temperature reaches 2000 K, the molecular hydrogen12 gets divided (dissociated) into two atoms.
This process uses energy that can not be used to maintain hydrostatic equilibrium. Result? The core becomes unstable and there is a second collapse.
After this collapse, returns hydrostatic equilibrium: now the core is smaller, and once again outer material falls into the core. This means another shock, but now luminosity does not rise as much as it did with the first shock (this is because now the surrounding cloud is not as optically thick - opaque - as before); nonetheless, the temperature rises albeit a constant luminosity, and at some point is high enough to burn deuterium, which is an atom of hydrogen with one proton and one neutron (plus one electron).
This type of process becomes the most favored process in the protostar to create energy (and become luminous), but initially, there is only a small amount of deuterium to burn: this means that luminosity drops.
When all the deuterium amount is burnt out, the track finishes with a decrease in temperature.
Ok, but do we see some protostars after all?
Well, the short answer is: it depends.
As we said earlier, the protostar core is shielded by dust. This makes it difficult to observe: astronomers need to observe in the infrared band. Moreover, these objects are relatively small and short-lived, so observing them is not trivial.
What comes next?
We will continue to follow the evolutionary track of the protostar up to the formation of the proper star. It will be a voyage full of funny names and original denominations, so prepare!
See you next time!
See you next time!13 (and if you liked subscribe!)
they are the particles that constitute light.
this is not the kind of dust you encounter in your house; it is made of chemical elements heavier than atomic hydrogen.
to give a scale is something like one-tenth of a trillionth times the density of distilled water. space IS empty.
that is, now the photon gets rapidly absorbed by dust, and can not freely wander towards the outer parts of the cloud.
pressure force = gravitational force.
1 AU is the (mean) distance that separates the Earth from the Sun, and it is approximately 150 million kilometers.
absolute magnitude is the quantity astronomers use to describe how a celestial object is luminous; it does not take into account how far away the object is but is indeed related to the luminosity the object has when we, here from Earth, observe it.
spectral types are the different kinds of shapes the spectra of the stars can have: you can visualize them as different skylines, whereas a single skyscraper or a mountaintop is unique for a spectral type.
this is called Stefan-Boltzmann law:
a blackbody can be thought of as an object that is very capable of transforming its energy in “temperature” (for the physicists reading this: forgive me).
that we don’t see in reality! Because the material surrounding the core blocks the light coming from the inner parts.
which is a molecule made up of two hydrogen atoms, viz.:
see, I told you!